TL;DR — There is more than one way to measure air quality! A variety of air quality monitoring technologies are in use today, including traditional reference-grade FRM/FEM monitors, mobile air quality sensors, stationary low-cost sensors, satellite monitoring technology, and alternative forms of monitoring — some projects have even successfully used moss to identify point sources of air pollution. When determining which type or types of monitoring technology will be the best fit for a monitoring project or program, it’s helpful to start by looking at the full range of available solutions. This allows you to explore the use cases for which each technology is best suited, along with any specific advantages and disadvantages. The increasing number of projects that leverage multiple technologies — a practice we refer to as Air Quality Monitoring 2.0 — presents exciting possibilities for the future of the global air quality monitoring space.
As air quality monitoring expands across the globe, so too do the types of monitoring technologies available. While in the United States, air quality monitoring began with the implementation of reference-grade equipment by the federal government, it has evolved to leverage alternative technologies such as mobile sensors, stationary low-cost sensors, air quality measurement conducted by satellites, and even the use of moss to detect air pollutants.
Each type of monitoring technology comes with its unique advantages and disadvantages. Each approach to air quality measurement also yields different data outcomes — different spatiotemporal resolution, accuracy, and more — and consequently can result in a very different understanding of the nature of air pollution.
As public demand for better air quality data grows, we will continue to see innovation in the air quality monitoring technologies that can help meet these needs. For example, smaller-scale air quality monitoring at local levels have become more prevalent as public awareness of environmental justice issues and other community-level air quality impacts have grown.
The most successful air quality monitoring projects are those that leverage the strengths of various technologies to paint the most complete picture of air pollution — this is where we see the future of air quality monitoring headed.
Traditional air quality monitoring using FRM and FEM equipment
Government reference-grade air quality monitors, categorized as either federal reference method (FRM) or federal equivalent method (FEM) monitors, represent the scientific standard for air quality monitoring. This equipment has strict standards for measurement performance and is typically used to support air quality decision-making, policymaking, and evaluation of attainment for regulatory standards at both state and federal levels.
FRM equipment is designed specifically to meet air quality measurement standards developed by regulatory bodies such as the United States Environmental Protection Agency (USEPA). FEM monitors may involve other types of technology but still must provide a comparable level of performance as assessed against official standards such as the USEPA’s.
Because of their high data quality, FRM and FEM monitors serve as the basis of comparison by which to judge other monitoring technologies such as low-cost sensors. Because important decision-making and regulatory action is based on air quality data from FRM and FEM devices, they have strict operating standards for parameters such as accuracy, precision, measurement range, and drift, among other aspects.

The high performance and data quality standards for FRM and FEM monitors set them up as the “gold standard” for air quality monitoring. Furthermore, their long operating lifetime — over ten years with proper maintenance and calibration — has allowed them to act as long-term instruments for federal air quality monitoring in the United States.
However, this technology is not without its drawbacks. Traditional FRM and FEM monitoring equipment come with a big price tag, with a typical purchase price of $15,000 to $40,000 per monitor. Properly operating the instrumentation often requires a temperature-controlled environment and routine calibration and maintenance require skilled technicians resulting in even higher operating costs; the annual cost to operate an FRM can often exceed the substantial purchase cost.
Because of their design and the infrastructure needed to support them — such as dedicated electrical power and data shelters to house the equipment — they tend to be inflexible when it comes to siting decisions. When it comes to deploying an air quality monitoring network, their high cost also means that fewer monitors can be implemented compared to the number of low-cost sensors or other alternative technologies that could be deployed at an equivalent cost. These barriers mean that air quality tends to be measured on a more regional scale, which can leave significant data gaps when it comes to understanding air quality at the local or neighborhood level.
Mobile air quality monitoring
As opposed to stationary air quality monitors, including both FRM/FEM monitors and stationary low-cost sensors, mobile air quality monitoring measures air quality across a city or region within a given timeframe — typically by mounting monitoring equipment on or inside of a car or other vehicle. This approach yields a spatially diverse, but temporally limited dataset to provide a snapshot of air quality across a region for a certain point in time.

One benefit of mobile air quality monitoring is the high spatial resolution at which measurement can occur. By using a “block by block” approach of gathering data at a large number of data points across a city, air quality measurements can be captured at locations not usually covered by a traditional FRM/FEM air quality monitoring network.
However, like other innovative monitoring technologies, mobile air quality monitors can have variable data quality that makes them inappropriate for regulatory use. The picture of air quality that comes from mobile monitoring can also be less informative than that of stationary monitoring networks.
Because the monitor is constantly moving locations, it could easily miss a pollution hotspot or local trend simply due to not being in the area at the time that an air pollution event occurred. While mobile monitoring can collect data at a higher spatial resolution, this does not guarantee that local air quality events are being captured in a meaningful way that allows you to understand air quality trends over time.
Stationary monitoring with low-cost air sensors
In terms of both spatial and temporal resolution, stationary low-cost air quality sensors fall in the middle-ground between traditional and mobile monitoring. The category is a broad one, though — a variety of low-cost sensors are available, with an equally wide range of performance.
Some low-cost sensors are marketed for both indoor and outdoor use and are best suited to home use by individual consumers. Other low-cost sensors — such as those we provide here at Clarity — place importance on not only the actual sensing technology but also the services that ensure its success. Calibrating the devices throughout their deployment, as well as integrating them with a cloud platform to provide scalability when building a network, ensures more accurate, effective, and user-friendly air quality monitoring.
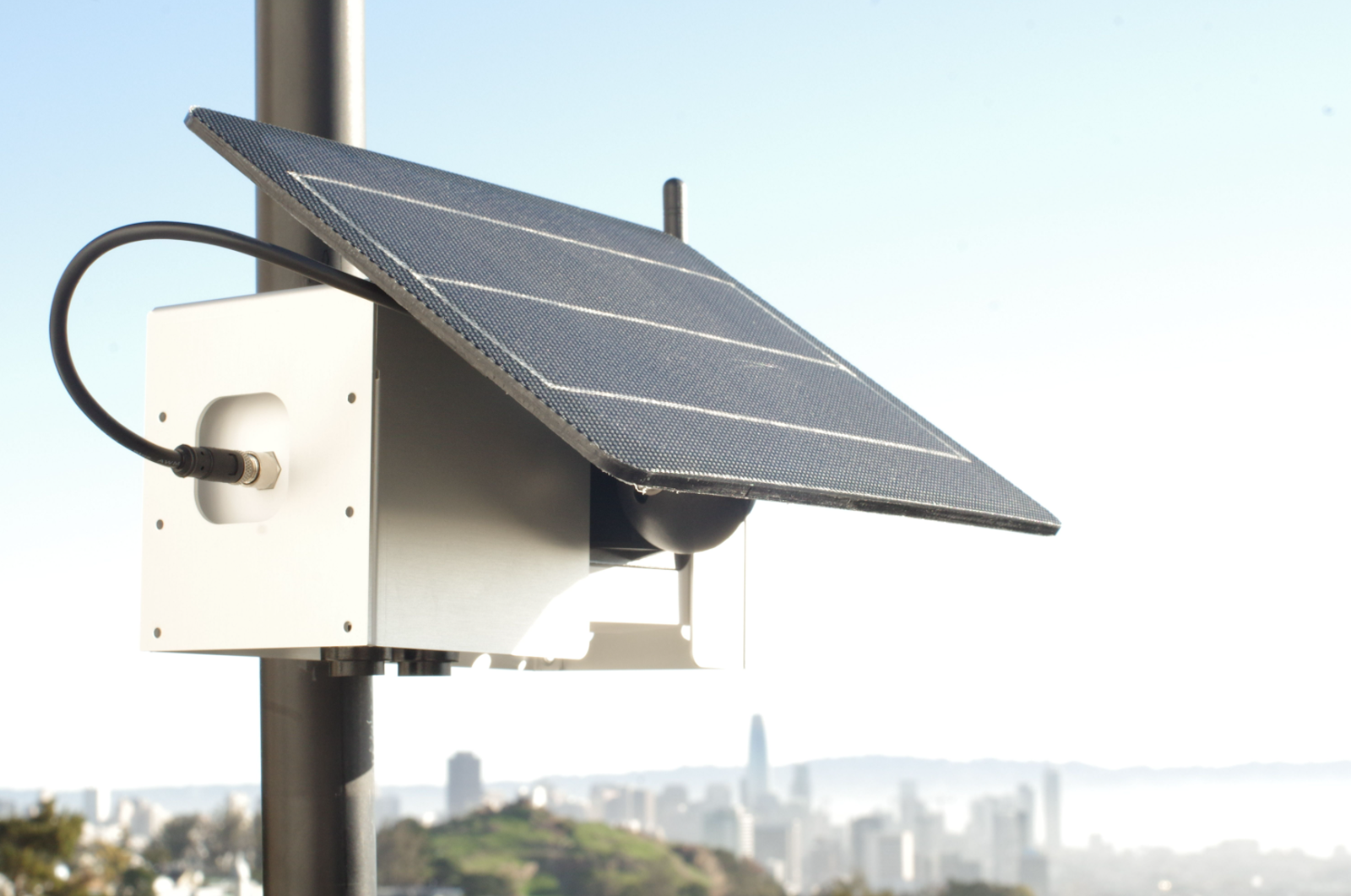
Apart from the data quality and associated services provided by different brands, low-cost sensors also differ in the infrastructure they require for operation — whether they rely on WiFi or dedicated electrical service, for example — which, in turn, affects the locations and conditions in which they can be deployed. When looking at deploying low-cost sensors in a variety of places across the globe, low-cost sensors which are self-sufficient and connect to the cloud via cellular for data collection can have real advantages.
As compared to mobile low-cost monitoring, stationary low-cost sensors can still provide high spatial data coverage without missing temporal trends. Flexible siting means that the sites that would benefit most from greater air quality monitoring coverage — such as those close to a pollution source or vulnerable population — can be prioritized during network design.
Stationary low-cost sensors’ lower purchase and operational costs, flexible deployment, and scalability make them useful for building sensor networks either in areas where a reference network does not exist, or to supplement reference-grade monitors in the region with more data points.
However, like mobile monitoring, stationary low-cost sensors can also vary in the quality of data they provide. Thus, it is crucial to assess the performance of the low-cost sensor you are interested in implementing as part of an air quality monitoring network. You can read our Guide to Accurate Particulate Matter Measurements with Air Sensors to learn more about the EPA’s release of performance targets for low-cost sensors and the important role that calibration plays in meeting these targets.
Air quality monitoring using satellites
As opposed to air quality monitors deployed at ground-level, air quality monitoring technology aboard satellites — such as NASA’s Moderate Resolution Imaging Spectroradiometer (MODIS) — can also be used to monitor pollution events and trends at a larger scale.
The technology used to monitor air quality from space is complex, dealing itself with the complexity of atmospheric chemistry. One type of measurement, aerosol optical depth (AOD), represents the density of aerosol particles by measuring the difference between solar radiation at the top of Earth’s atmosphere compared to that which reaches its surface. A larger AOD measurement occurs when there are more particles in the air and, thus, less radiation that can be detected at the surface. In general, satellite-based air quality monitoring operates by looking at how sunlight is scattered by ambient particulates, such as PM2.5; however, this process can easily be disrupted by weather conditions, properties of the pollutant particles, atmospheric mixing, and other factors. Dependence on sunlight also means this monitoring cannot operate during a cloudy day.
In many applications, satellite air quality monitoring data is fused with ground-based measurements to lend a “bigger picture” view of air quality. For example, combining a variety of data points from the satellite monitor itself — such as AOD with cloud optical thickness or wind speed and direction — with ground-level air quality index (AQI) and knowledge about recent fire locations can contribute to a more complete and accurate forecast of air quality during wildfire events.
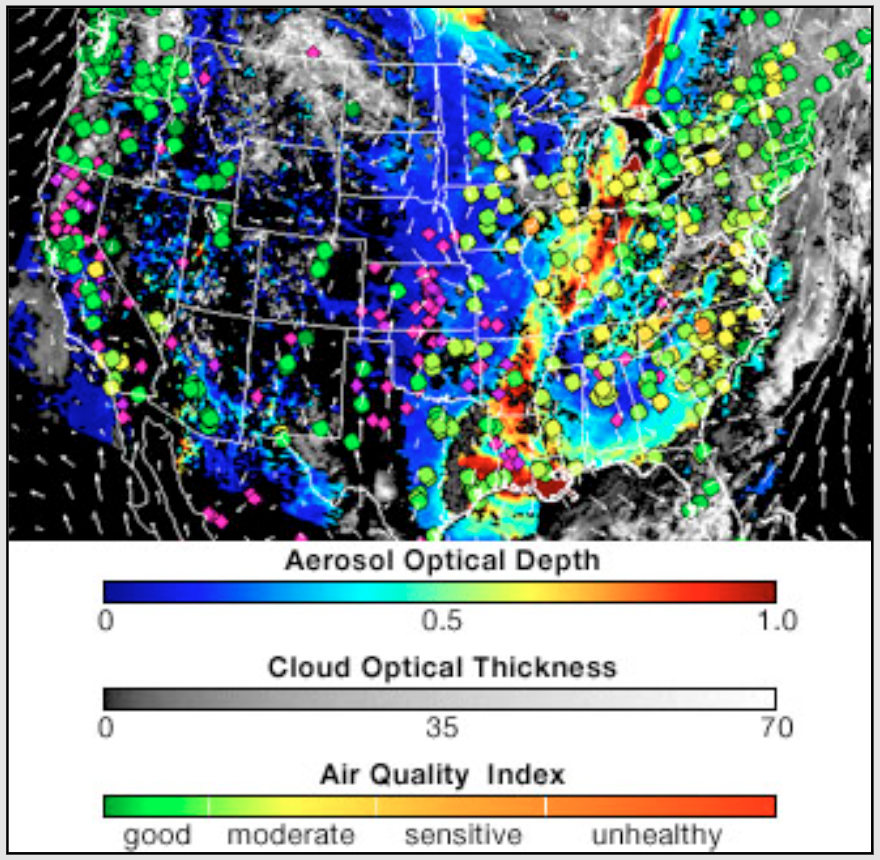
Another type of air quality monitoring instrument aboard satellites is known as the ozone monitoring instrument (OMI) or TROPOspheric Monitoring Instrument (TROPOMI) and is used by NASA. By measuring the intensity of reflected sunlight at various wavelengths, and detecting the absorption features of various atmospheric trace gases, they can measure not only ozone but also substances like nitrogen dioxide, sulfur dioxide, and formaldehyde. This technology can observe the entire Earth in a single day and track changes in global air quality over time.
However, like other satellite air quality monitoring technology, they cannot collect data during cloudy conditions, since it functions using sunlight reflected back to space. Monitoring air quality using satellite technology can help fill in the gaps left behind by ground-based monitors, including over remote regions or bodies of water, in countries where ground-level monitors have not yet been deployed or which lack the infrastructure, and in any region where an existing monitoring network is sparse or nonexistent.
This bird’s-eye view also means that air pollution can be tracked as it moves around the globe with much greater geographical coverage than other monitoring technologies. They also act as a relatively inexpensive source of supplemental air quality data.
However, satellite air quality monitoring currently operates at a fairly low temporal and spatial resolution compared to ground-based monitoring and at variable levels of accuracy due to calibration biases. Satellite-based models also must be ground-truthed using ground-based monitoring, so they will likely continue to support existing monitoring technologies rather than replace ground-based monitoring.
Other approaches to air quality monitoring: research using moss in Oregon
Of course, there are ways to characterize air quality without the use of any instruments whatsoever —while air pollution is often invisible, in certain cases we can even use our sense of sight or smell to surmise whether air quality is good or poor.
One interesting alternative approach to monitoring air quality has been explored in the Pacific Northwest region of the United States. In 2016, researchers in Portland, Oregon conducted a study to see whether certain types of moss on trees could be used to evaluate air quality. Their research detected levels of toxic heavy metals, such as cadmium and arsenic, that greatly surpassed the state’s limits. Researchers used this information to create maps of where state regulators could site air quality sensors in Portland as well as map hotspots of toxic heavy metal levels near glass factories in the city.
Plants like moss and lichen have been long used to test air quality in forests, but this was the first time that this was successfully conducted in an urban environment. This alternative method of air quality monitoring is possible because moss acts as a natural air pollution monitor — it absorbs gases from its environment and can reflect subtle changes in the air.
Using moss for air quality monitoring is relatively inexpensive compared to other monitoring methods: for $20,000, more than 300 locations across a city can be tested using moss sampling, as compared to the $150,000 price tag of running a reference-grade single air quality monitor for a year.
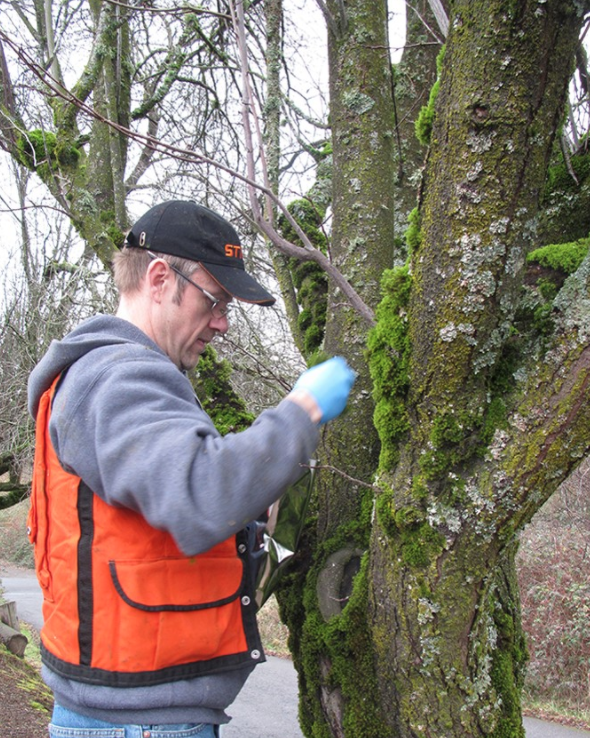
With this lower cost comes higher data resolution — especially given how prevalent moss and lichen are in Portland. While a localized air quality event may leave a plume that only goes for a couple of miles, and an air quality monitor may not be located within this range, there is likely to be moss on trees or rocks nearby from which air quality information can be collected, giving insight into the state of air quality in that location at that specific time.
While this alternative monitoring method is exciting, it also cannot be used alone to measure air quality — given the lack of precision with this method, it is best used as an indicator and not as a data source for quantifying air pollutant concentrations. Instead, using moss to collect air quality data can be a supplemental part of existing air quality monitoring networks in a location. This approach can also only be used in geographic regions where moss or lichen occurs naturally, and thus does not apply to air quality monitoring in many regions of the world.
Air Quality Monitoring 2.0: Using the full range of air monitoring technologies to better understand air pollution
Recent developments in air quality monitoring technologies present exciting possibilities for the future of air quality management. Both traditional and new types of monitoring technologies come with their own strengths, weaknesses, and best-fit use cases — and the most successful air quality monitoring programs of the future will be those that leverage the strengths of each technology.
While traditional FRM/FEM monitoring equipment provides high-quality data, it can also be very costly, inflexible, and difficult to site. Mobile air quality monitors are more inexpensive and can capture a high volume of data, but their mobile nature means that incidents of heightened air pollution at specific sites can be missed. Air quality monitors aboard satellites can help give a big-picture view of air pollution over a wider geographic area, but their spatial and temporal resolution remains low at their current level of development. Other alternative methods of air pollution assessment, such as using moss or lichen, can provide insightful supplemental data, but it cannot be used alone nor in a wide range of climatic regions.
While stationary low-cost sensors can vary in data quality depending on the performance of the specific sensor and the calibration methods applied, they combine the flexibility of mobile monitoring with the advantages of stationary monitoring — making them a great option to cost-effectively add measurement points to air quality monitoring networks.
Low-cost sensors can be implemented both as part of a hybrid air quality monitoring network in conjunction with reference-grade federal monitors or as a standalone solution in places where no monitoring network exists. Though LCS do not provide regulatory-level data, choosing a high-quality sensor technology provider that understands the importance of rigorous calibration and colocation processes helps to ensure the most accurate data possible with this technology.
To learn how to establish your own hybrid Air Quality Monitoring 2.0 network, you can download our Guide to Leveraging Low-Cost Sensors for Air Quality Monitoring 2.0.